3.1 Pure Substances
A substance with a fixed chemical composition is called a pure substance. For example, water, nitrogen, helium, and carbon dioxide are all pure substances.
A mixture of various chemical elements or compounds also qualifies as a pure substance as long as the mixture is homogeneous. For example, air is a mixture of several gases, but it is often considered to be a pure substance because it has a uniform chemical composition.
However, a mixture of oil and water is not a pure substance because oil is not soluble in water, it will collect on top of the water, forming two chemically dissimilar regions.
A mixture of two or more phases of a pure substance is still a pure substance as long as the chemical composition of all phases is the same. A mixture of ice and liquid water, for example, is a pure substance because both phases have the same chemical composition.
A mixture of liquid air and gaseous air, however, is not a pure substance as the composition of liquid air is different from the composition of gaseous air chemically, and different components in air condense at different temperatures at a specified pressure.
Conclusion
Anything is called to be called pure substance if
- a substance with a fixed chemical composition
- a homogeneous mixture of various chemical elements with uniform composition chemically.
- a mixture of two or more phases of a pure substance with the same chemical composition for all phases.
3.2 Phase of Pure Substance
A phase is identified as having a distinct molecular arrangement that is homogeneous throughout and separated from the others by easily identifiable boundary surfaces.
Under different conditions, each may appear in a different phase. For example,
- Carbon may exist as graphite or diamond in the solid phase.
- Helium has two liquid phases.
- Iron has three solid phases.
- Ice may exist at seven different phases at high pressure.
3.2.1 Molecular activity in a different state of Matter
Intermolecular bonds are strongest in solids and weakest in gases. One reason is that molecules in solids are closely packed together whereas in gases they are separated by relatively large distances.
Even though the molecules in a solid cannot move relative to each other, they continually oscillate about their equilibrium positions. The velocity of the molecules during these oscillations depends on the temperature. At sufficiently high temperatures, the velocity of the molecules may reach a point where the intermolecular forces are partially overcome and groups of molecules break away which is the beginning of the melting process.
In liquid, the molecules are no longer at fixed positions relative to each other and they can rotate and translate freely.
In the gas phase, the molecules are far apart from each other, and a molecular order is non-existent. Molecules in the gas phase are at a considerably higher energy level than they are in the liquid or solid phases. Therefore, the gas must release a large amount of its energy before it can condense or freeze.
Particularly at low densities, the intermolecular forces are very small, and collisions are the only mode of interaction between the molecules.
3.3 Phase Change Process of Pure Substance
Let's study the phase-change process of any pure substance at different temperatures while keeping the pressure constant (say a closed system with one movable wall through a piston filled with water at 1 atm and room temperature).
State 1 and 2: Compressed and Saturated Liquid
State 1: Under these conditions, water exists in the liquid phase, and it is called a compressed liquid, or a subcooled liquid, meaning that it is not about to vaporize. As the temperature rises, the liquid water expands slightly, and so its specific volume increases. To accommodate this expansion, the piston moves up slightly. The pressure in the cylinder remains constant at 1 atm during this process since it depends on the outside barometric pressure and the weight of the piston.
State 2: As more heat is transferred, the temperature keeps rising until it reaches 100°C. At this point, water is still a liquid, but any heat addition will cause some of the liquid to vaporize. That is, a phase-change process from liquid to vapor is about to take place. A liquid that is about to vaporize is called a saturated liquid.
At a given pressure, the temperature at which a pure substance changes phase is called the saturation temperature (Tsat).
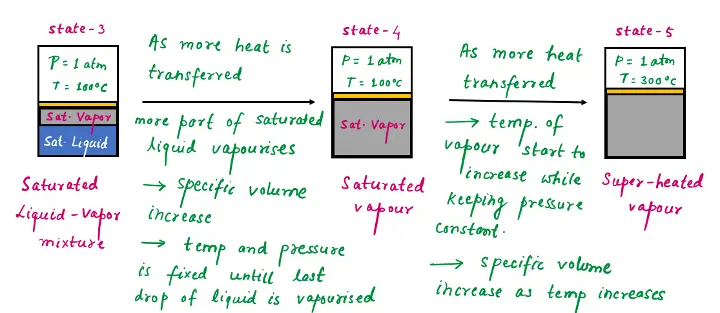
State 3,4, and 5: Saturated Vapor and Superheated Vapor
Once boiling starts, the temperature stops rising until the liquid is completely vaporized. The temperature will remain constant during the entire phase-change process if the pressure is held constant.
Saturated Liquid-Vapor Mixture
State 3: Midway through the vaporization line, the cylinder contains equal amounts of liquid and vapor. As we transfer heat, the vaporization process continues until the last drop of liquid is vaporized.
State-4: At this point, the entire cylinder is filled with vapor that is on the borderline of the liquid phase. Any heat loss from this vapor will cause some of the vapor to condense (phase change from vapor to liquid). A vapor that is about to condense is called a saturated vapor.
A substance at states between 2 and 4 is called a saturated liquid-vapor mixture since the liquid and vapor phases coexist in equilibrium at these states.
State-5: Once the phase-change process is completed (liquid into vapor completely), then further transfer of heat results in an increase in both the temperature and the specific volume. Therefore, water at state 5 is a superheated vapor.
For easy understanding of the whole constant-pressure phase-change process, can be illustrated on a T-v diagram.
Saturation Temperature and Saturation Pressure
The temperature at which water starts boiling depends on the pressure; therefore, if the pressure is fixed, so is the boiling temperature.
At a given pressure, the temperature at which a pure substance changes phase is called the saturation temperature (Tsat).
At a given temperature, the pressure at which a pure substance changes phase is called the saturation pressure (Psat).
The amount of energy absorbed or released during a phase-change process is called the latent heat. The amount of energy absorbed during melting is called the latent heat of fusion and is equivalent to the amount of energy released during freezing.
The amount of energy absorbed during vaporization is called the latent heat of vaporization and is equivalent to the energy released during condensation.
During a phase-change process, the latent heats depend on the temperature or pressure, while pressure & temperature are dependent properties such as
A plot of a graph between Tsat versus Psat is called a liquid-vapor saturation curve. A curve of this kind is characteristic of all pure substances.
As, from the above graph of the liquid-vapor saturation curve, Tsat increases with Psat. Thus, a substance at a higher pressure boils at higher temperatures. Based on this line, phenomena of many real-life activities can be explained such as:
-
In the kitchen, higher boiling temperatures mean shorter cooking times and energy savings.
- It takes longer to cook at higher altitudes than it does at sea level because of low atmospheric pressure, and thus, the boiling temperature of water, decreases with elevation.
Some Consequences of Tsat and Psat Dependence
A substance at a specified pressure boils at the saturation temperature corresponding to that pressure. This phenomenon allows us to control the boiling temperature of a substance by simply controlling the pressure.
3.4 Property Diagram for Phase-Change Process
3.4.1 T-V Diagram
Firstly create high pressure inside the cylinder by adding weight on top of piston (let us take 1Mpa). Water has a smaller specific volume at this pressure than it does at 1 atm pressure.
As heat is transferred to the water at this new pressure, the process follows a path that looks very much like the process path at 1 atm pressure. But there are two differences between these two processes-
-
Water (pressure=1 Mpa) will start boiling at a higher temperature (179 C) instead of 100C.
- Second, the specific volume of the saturated liquid is larger and the specific volume of the saturated vapor is smaller than the corresponding values at 1 atm pressure.
Critical Point
As a result, the horizontal line that connects the saturated liquid and saturated vapor states becomes much shorter. Hence, as the pressure is increased further, this saturation line continues to shrink and will reach a point at a certain pressure (22.06 MPa in the case of water) where saturated liquid and saturated vapor can’t be differentiated. That point is called the Critical Point.
A critical point is defined as the point at which the saturated liquid and saturated vapor states are identical.
The temperature, pressure, and specific volume of a substance at the critical point are called the critical temperature (Tcr), critical pressure (Pcr,) and critical specific volume (Vcr), respectively.
There is no distinct phase change process at pressures above the critical pressure. Instead, the specific volume of the substance continually increases, and at all times there is only one phase present. Above the critical state, no line separates the compressed liquid region and the superheated vapor region.
However, it is customary to refer to the substance as superheated vapor at temperatures above the critical temperature and as compressed liquid at temperatures below the critical temperature.
3.4.2 P-V Diagram
Consider again a piston-cylinder device that contains liquid water at 1 MPa and 150°C. Water in this state exists as a compressed liquid.
Now the weights on top of the piston are removed one by one so that the pressure inside the cylinder decreases gradually. The water is allowed to exchange heat with the surroundings so its temperature remains constant. As the pressure decreases, the volume of the water increases slightly.
Saturation-Pressure Value (Phase-change process start)
When the pressure reaches the saturation-pressure value at the specified temperature (0.4762 MPa), the water starts to boil. During this vaporization process, both the temperature and the pressure remain constant, but the specific volume increases.
Once the last drop of liquid is vaporized, further reduction in pressure results in a further increase in specific volume.
When the process is repeated for other temperatures, similar paths are obtained for the phase-change processes. Connecting the saturated liquid and the saturated vapor states by a curve, we obtain the P-v diagram of a pure substance.
Precautions
During the phase-change process, we did not remove any weights. Doing so would cause the pressure and therefore the temperature to drop [since Tsat f (Psat)], and the process would no longer be isothermal
3.5 Property Tables
For most substances, the relationships among thermodynamic properties are too complex to be expressed by simple equations. Therefore, properties are frequently presented in the form of tables.
A separate table is prepared for each region of interest, such as the superheated vapor, compressed liquid, and saturated (mixture) regions.
3.5.1
Saturated Liquid and Saturated Vapor States
Subscript f is used to denote the properties of a saturated liquid, and subscript g is used to denote the properties of saturated vapor.
Subscript fg, which denotes the difference between the saturated vapor and saturated liquid values of the same property.
The quantity Hfg is called the enthalpy of vaporization (or latent heat of vaporization). It represents the amount of energy needed to vaporize a unit mass of saturated liquid at a given temperature or pressure. It decreases as the temperature or pressure increases and becomes zero at the critical point.
If saturated pressure is given in question instead of saturated temperature, we will use A-5 table as a pressure table for saturated water. There will be different property values of saturated liquid and vapor at saturated temperature corresponding to the given pressure value.
3.5.2
Saturated Liquid–Vapor Mixture
During a vaporization process, a substance contains a mixture of saturated liquid and saturated vapor. To analyze this mixture properly, we need to know the proportions of the liquid and vapor phases in the mixture by defining a new property called the quality x as the ratio of the mass of vapor to the total mass of the mixture.
During the vaporization process, only the amount of saturated liquid or vapor changes, not its properties. The properties of saturated mixture will be the average properties of the saturated liquid-vapor mixture under consideration as two phases are mixed well, forming a homogeneous mixture.
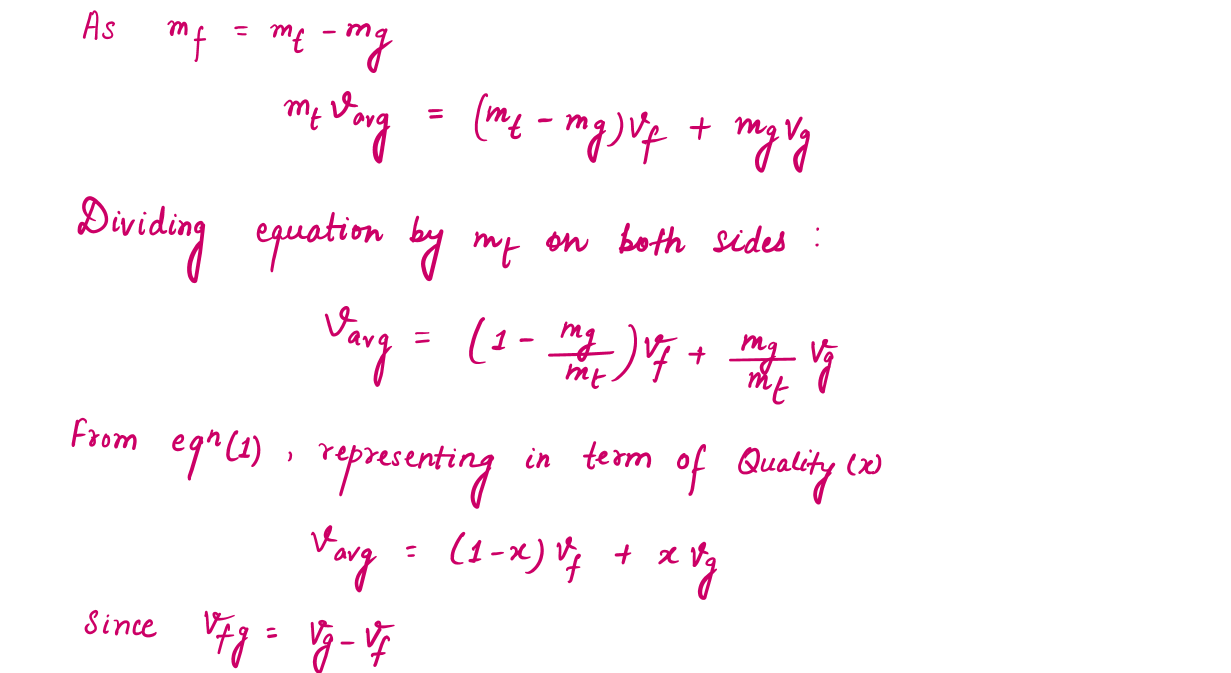
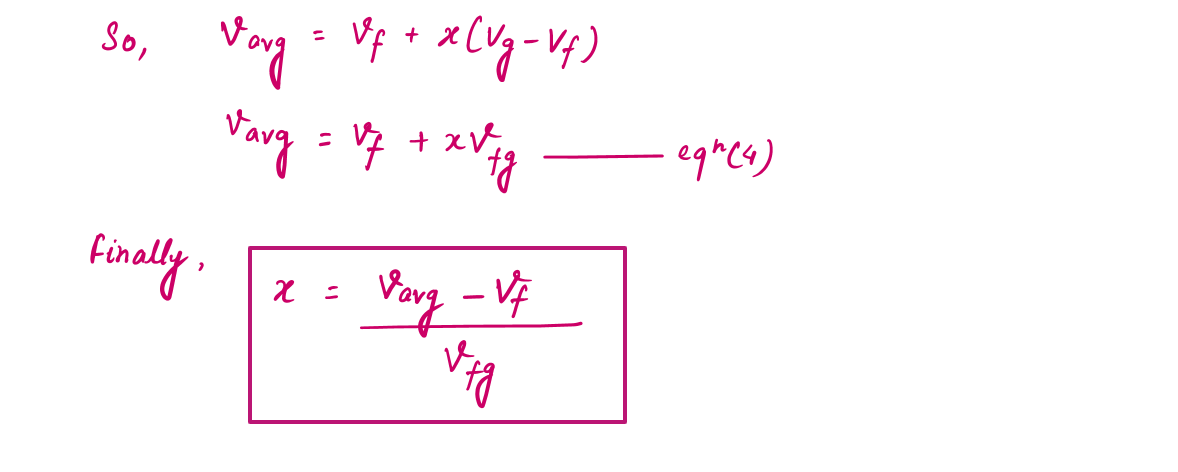
The Significance of Value of X (Quality) in P-T and P-V Diagram
Based on this equation, quality can be related to the horizontal distances on a P-v or T-v diagram.
At a given temperature or pressure, the numerator of X is the distance between the actual state and the saturated liquid state, and the denominator is the length of the entire horizontal line that connects the saturated liquid and saturated vapor states. A state of 50 percent quality lies in the middle of this horizontal line.
Defining Internal Energy and Enthalpy through Properties of Saturated Liquid-vapor Mixture
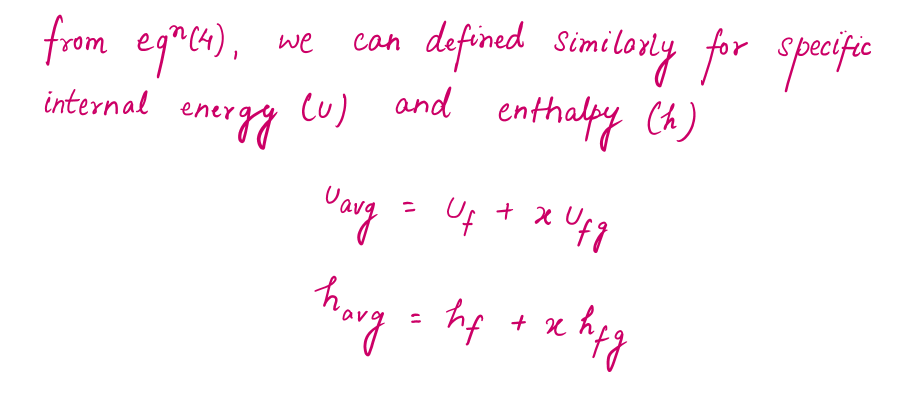
3.5.3 Superheated Vapor
In the region to the right of the saturated vapor line and at temperatures above the critical point temperature, a substance exists as superheated vapor. Since the superheated region is a single-phase region (vapor phase only), temperature and pressure are no longer dependent properties and they can conveniently be used as the two independent properties in the tables.
If we compare it to saturated vapor, a superheated vapor is characterized by
- Lower pressures (P < Psat at a given T)
- Higher temperature (T > Tsat at a given P)
- Higher specific volumes (v > vg at a given P or T)
- Higher internal energies (u > ug at a given P or T)
- Higher enthalpies (h > hg at a given P or T)
3.5.4 Compressed Liquid
Generally, we lack data on compressed liquid at extremely high pressure (more than 50 Mpa) or extremely lower pressure ( less than 5 Mpa) in the property table. One reason for the lack of compressed liquid data is the relative independence of compressed liquid properties from pressure. Variation of properties of compressed liquid with pressure is very mild. Increasing the pressure 100 times often causes properties to change less than 1 percent.
In the absence of compressed liquid data, a general approximation is to treat compressed liquid as saturated liquid at the given temperature because the compressed liquid properties depend on temperature much more strongly than they do on pressure.
In general, a compressed liquid is characterized by
- Higher pressures (P > Psat at a given T)
- Lower temperatures (T < Tsat at a given P)
- Lower specific volumes (v < vf at a given P or T)
- Lower internal energies (u < uf at a given P or T)
- Lower enthalpies (h < hf at a given P or T)
Remembering Part
"Compressed liquid have pressure more than saturated pressure at given temperature."
Rest, you don't need to cramp rest of those condition. One condition is enough to remember as these condition are relative for compressed liquid and superheated vapor.